A few months ago, I went to a creative origami lunchtime session organised by some lovely people at $WORK
. I’d done origami a bit when I was younger, but mostly just frogs and cranes, which have since helped me while away the hours when invigilating exams. However, at this lunchtime session I was shown how to make modular origami. This involves making lots of (generally quite simple) origami parts, and then slotting them together to make larger structures.
![Sonobe origami assembly [CC-BY-SA-3.0 Steve Cook]](https://www.polypompholyx.com/wp-content/uploads/2017/01/sonobe_origami_assembly-300x126.png)
I went home with a simple 12-unit Sonobe ball that afternoon and was very pleased with myself.
![Cumulated octadedron (sonobe) [CC-BY-SA-3.0 Steve Cook]](https://www.polypompholyx.com/wp-content/uploads/2017/01/cumulated_octadedron_sonobe-285x300.jpg)
Entry-level modular origami: the 12-unit Sonobe ball. Mathematically, it’s a cumulated octahedron; practically, it’s 12 sheets of square paper and about 1 hour of your time.
Things have rather escalated from there.
![Assorted modular origami [CC-BY-SA-3.0 Steve Cook]](https://www.polypompholyx.com/wp-content/uploads/2017/01/assorted_modular_origami-300x243.jpeg)
Between running some of these lunchtime sessions myself now, and being asked on several occasions on Twitter about how I make the pretty things I keep tweeting, I thought it’d be useful to put together a quick guide (or a link-farm, at least)..
Sonobe units are very easy to fold, quite forgiving, and can be used to make a cube (6 units), a cumulated octahedron (12 units), a cumulated icosahedron (30), and a kind-of truncated icosahedron (90, basically a spiky football). They’re a pretty good introduction to the general principles:
![Assorted (sonobe) [CC-BY-SA-3.0 Steve Cook]](https://www.polypompholyx.com/wp-content/uploads/2017/01/assorted_sonobe-300x190.jpg)
Sonobe family: 90, 30, 12, 6 and 3 units. The 3-unit one is a trigonal bipyramid but barely counts! These have all been made with the slightly modified unit mentioned below. The 90 units one is the biggest Sonobe that’s really worth making IMHO: about 3 hours’ work
The 30-unit ball has the symmetries of an
icosahedron (or
dodecahedron). Once you’ve learnt how to construct that object in Sonobe modules, you’ve essentially learnt how to construct any 30-unit modular origami ball: they mostly involve slotting 30 edge-units into groups of three to form the 12 pentagonal faces of a dodecahedron (or equivalently/alternatively, slotting them into groups of five to form the 20 triangular faces of an icosahedron – the difference is mostly one of perspective).
![Cumulated icosahedron (sonobe) [CC-BY-SA-3.0 Steve Cook]](https://www.polypompholyx.com/wp-content/uploads/2017/01/cumulated_icosahedron_sonobe-300x284.jpg)
Cumulated icosahedron made of Sonobe units: 30 sheets of paper, and – if you’ve got the hang of the 12-unit ball – still only 1 hour of your time
There are lots of variations on the Sonobe unit you can (re)invent, by adding back-folds that expose the other side of the paper, or that make the tabs narrower than the pockets, giving a more intricate look.
![Cumulated icosahedron (sonobe variant) [CC-BY-SA-3.0 Steve Cook]](https://www.polypompholyx.com/wp-content/uploads/2017/01/cumulated_icosahedron_sonobe_variant-300x282.jpg)
Cumulated icosahedron made of slightly modified Sonobe units
Although the 90-unit structure is quite stable, the next one up (270 units) tends to sag under its own weight over time, but by that point it felt like a right of passage to make one.
![270 (sonobe colourchange) [CC-BY-SA-3.0 Steve Cook]](https://www.polypompholyx.com/wp-content/uploads/2017/01/270_sonobe_colourchange-300x300.jpg)
9 hours construction, plus some planning. This uses duo paper, which is coloured on both sides, and a modified Sonobe unit that has a reverse fold to expose the other side of the paper in each module.
The Sonobe units can also be assembled inside-out to make inwardly cumulated polyhedra…
![Cumulated postive negative icosahedra (sonobe) [CC-BY-SA-3.0 Steve Cook]](https://www.polypompholyx.com/wp-content/uploads/2017/01/cumulated_postive_negative_icosahedra_sonobe-300x164.jpg)
Getting the last few units in on the inverted ball (left) is tricky.
…and they can also be assembled in pairs and then assembled into a
spiked pentakis dodecahedron...
![Pentakis dodecahedron (sonobe colourchange pairs) [CC-BY-SA-3.0 Steve Cook]](https://www.polypompholyx.com/wp-content/uploads/2017/01/pentakis_dodecahedron_sonobe_colourchange_pairs-300x294.jpg)
Pentakis dodecahedron, with a reverse-fold Sonobe unit that shows the other side of the paper.
…and other structures.
![Rhombic triacontahedron (sonobe colourchange pairs) [CC-BY-SA-3.0 Steve Cook]](https://www.polypompholyx.com/wp-content/uploads/2017/01/rhombic_triacontahedron_sonobe_colourchange_pairs-300x283.jpg)
The site above describes this as a rhombic triacontahedron, but I’m pretty sure it isn’t. I’m not sure what it actually is though. Has both colour-change and the units are assembled ‘inside out’ to make it inwardly cumulated.
The next unit I tried was the Penultimate edge unit (
attributed to Robert Neal), which can be used to
make a wireframe dodecahedron, as demonstrated by Matt Parker, the stand-up mathematician. Other variations of this subunit can be used to make pretty much any other wireframe polyhedron.
![Dodecahedron (penultimate) [CC-BY-SA-3.0 Steve Cook]](https://www.polypompholyx.com/wp-content/uploads/2017/01/dodecahedron_penultimate-300x296.jpg)
Dodecahedron. I was trying to use up the boring coloured paper on this one, but I quite liked the result in the end!
Thomas Hull’s
PhiZZ edge unit makes similar wireframe structures, but the modules fit together more tightly and the resulting structures are much more robust than you get with the penultimate modules.
![Truncated icosahedron (phizz) [CC-BY-SA-3.0 Steve Cook]](https://www.polypompholyx.com/wp-content/uploads/2017/01/truncated_icosahedron_phizz-300x225.jpg)
Truncated icosahedron – this is basically the shape of a football (12 pentagons, surrounded by hexagons) and of some viral capsids too.
You can also make colour-change variants using the technique shown in
Lewis Simon’s decoration boxes.
![Dodecahedron (phizz colourchange) [CC-BY-SA-3.0 Steve Cook]](https://www.polypompholyx.com/wp-content/uploads/2017/01/dodecahedron_phizz_colourchange-297x300.jpg)
Dodecahedron made from PHiZZ units with a colour-change.
For structures based on dodecahedra/icosahedra and made from edge-units, you can always get away with using just three colours and never have two of the same colour pieces touching. This is because you can draw a
Hamiltonian circuit on a dodecahedron: that is a path from vertex to vertex that only visits each vertex once, and which comes back to where it started. You can represent this in 2D on a
Schlegel diagram.
![Hamiltonian circuit through a dodecahedron [CC BY-SA 3.0 Tomruen/Steve Cook]](https://www.polypompholyx.com/wp-content/uploads/2017/01/hamiltonian_circuit_dodecahedron-300x290.png)
Hamiltonian circuit through the Schlegel diagram of a dodecahedron [CC BY-SA 3.0 original by Tomruen, modified by Steve Cook]. The red and purple edges form the Hamiltonian circuit; the grey edges are what is left over. You’ll notice that every vertex has one of each of the three coloured edges. The diagram is a projection of a dodecahedron: imagine taking a wireframe of the dodecahedron and shining a torch through it: the Schlegel diagram is the 2D shadow this 3D polyhedron casts on the wall. It’s fairly easy to work out which edge in the 2D diagram correspond to which edge in the thing you’re building.
If you colour alternate edges of the Hamiltonian circuit in two of your chosen colours, and the rest of the edges in the third, then you’ll avoid having any colour-clashes. I only learnt this after I started making these structures, so not all of them have this optimal colouring! The same 3-colour rule is true for the other Platonic solids, and also for
the truncated icosahedron.
Francesco Mancini’s star-holes kusudama uses a similar module to the PHiZZ, but with a little back-bend that gives a nice 3D star effect. This one is a dodecahedron-shaped (30 units), but a 90-unit truncated icosahedron should also be possible.
![Star holes dodecahedron [CC-BY-SA-3.0 Steve Cook]](https://www.polypompholyx.com/wp-content/uploads/2017/01/star_holes_dodecahedron-300x297.jpg)
Star-holes dodecahedron.
UPDATE: yes, it is possible 🙂
![Star-holes truncated icosahedron [CC-BY-SA-3.0 Steve Cook]](https://www.polypompholyx.com/wp-content/uploads/2017/04/star_holes_truncated_icosahedron-300x225.jpg)
Star-holes truncated icosahedron
Lewis Simon and Bennett Arnstein’s triangle edge unit can be used to make very nice patchtwork tetrahedra, octahedra and icosahedra.
![Icosahedron (triangle edge) [CC-BY-SA-3.0 Steve Cook]](https://www.polypompholyx.com/wp-content/uploads/2017/01/icosahedron_triangle_edge-300x285.jpg)
Icosahedron.
They’re a bit fiddly to put together but are very robust once constructed. A similar patchwork effect for the dodecahedron can be achieved with M. Mukhopadhyay’s
umbrella module; Sonobe units can be used to make analogous Battenberg-cake style cubes.
![Assorted (triangle edge sonobe umbrella) [CC-BY-SA-3.0 Steve Cook]](https://www.polypompholyx.com/wp-content/uploads/2017/01/assorted_triangle_edge_sonobe_umbrella-300x177.jpg)
Battenberg-cake Platonic solids. The dodecahedron is made from umbrella units; the cube from Sonobe. The tetrahedron, octahedron and icosahedron are all made from triangle edge modules.
The
simple isosceles triangle unit (attributed variously to M. Mukhopadhyay, Jeannine Mosely and Roberto Morassi) can be used to make small and great stellated dodecahedra.
![Small and great stellated dodecahedron (isosceles) [CC-BY-SA-3.0 Steve Cook]](https://www.polypompholyx.com/wp-content/uploads/2017/01/small_and_great_stellated_dodecahedron_isosceles-300x163.jpg)
Great (left) and small (right) stellated dodecahedra.
The
small stellated dodocahedron is particularly pleasing and makes a fairly robust decoration if made of foil-backed paper.
![Small stellated dodecahedron (isosceles) [CC-BY-SA-3.0 Steve Cook]](https://www.polypompholyx.com/wp-content/uploads/2017/01/small_stellated_dodecahedron_isosceles-300x276.jpg)
Xmas decs
The great stellated dodecahedron can be made from the same subunit, but is tricker to construct because a tab has to curl around into a pocket that is partly inside the next tab round. I used needle-nosed forceps to construct this, and I’m still not terribly happy with the result.
The opposite is true for Paolo Bascetta’s star module, which makes a great great stellated dodecahedron, but a rather *eh* small stellation. This module needs duo paper (i.e. paper that is coloured on both sides) for best effect.
![Small and great stellated dodecahedron (star) [CC-BY-SA-3.0 Steve Cook]](https://www.polypompholyx.com/wp-content/uploads/2017/01/small_and_great_stellated_dodecahedron_star-300x138.jpg)
Great (left) and small (right) stellated dodecahedra.
Dave Mitchell’s Electra module can be used to make a icosidodecahedron: it’s unusual in that each module corresponds to one vertex of the structure: the edge units described up to this point combine together to make each vertex.
![Icosidodecahedron (electra) [CC-BY-SA-3.0 Steve Cook]](https://www.polypompholyx.com/wp-content/uploads/2017/01/icosidodecahedron_electra-295x300.jpg)
Icosidodecahedron made from Electra modules
I’m not that happy with my
Void kusudama (Tadashi Mori): I should have used duo paper, but it was really tricky to put together. Maybe one day. It’s one of the few structures here that is back to the original octahedral/cubic 12-unit structure. I’m not sure the 30-unit version would be stable.
![Octahedral void [CC-BY-SA-3.0 Steve Cook]](https://www.polypompholyx.com/wp-content/uploads/2017/01/octahedral_void-300x289.jpg)
Octahedral void
UPDATE: Yeah, I don’t think the 30-unit version is do-able. I think the units are too wide to actually fit into an icosahedron: I couldn’t even manage it with glue, so I don’t think it’s just a stability issue. However, I did do a better 12-unit version, with duo paper and a little reverse fold on the outer edge to expose the second colour properly, which I’m quite pleased with:
![Octahedral void (modified) [CC-BY-SA-4.0 Steve Cook]](https://www.polypompholyx.com/wp-content/uploads/2017/04/octahedral_void_modified-300x298.jpg)
Octahedral void (modified)
Tomoko Fusè’s little turtle modules are extremely flexible: they can be used to make pretty much any polyhedron that is made of regular polygons. However, because the flaps are only one paper layer thick, they don’t fit together terribly tightly, so I’ve only found them robust enough to make smaller structures without the help of glue. However, with glue, I’ve made a
rhombicosidodecahedron, which is cool because it is built of pentagons, triangles
and squares (all of the polygons found in the Platonic solids)…
![Rhombicosidodecahedron (little turtle) [CC-BY-SA-3.0 Steve Cook]](https://www.polypompholyx.com/wp-content/uploads/2017/01/rhombicosidodecahedron_little_turtle-300x300.jpg)
The impossible-to-spell rhombicosidodecahedron.
…and also a
pair of snub-cubes, which are even more interesting as the snub-cube has
two non-superimposable mirror images, like hands, amino acids and amphetamines.
![Snubcubes (little turtle) [CC-BY-SA-3.0 Steve Cook]](https://www.polypompholyx.com/wp-content/uploads/2017/01/snubcubes_little_turtle-300x225.jpg)
Snubcubes: left- and right-handed enatiomorphs.
I found
Maria Sinayskaya Etna kusudama in
Meenakshi Mukerji’s Exquisite Modular Origami book. It’s a really pretty model, and robust once it’s assembled, but it can be a bit fally-aparty during construction: I used very small clothes pegs to hold it together as I was making it.
![Etna kusudama [CC-BY-SA-3.0 Steve Cook]](https://www.polypompholyx.com/wp-content/uploads/2017/01/etna_kusudama-300x285.jpg)
Etna kusudama.
Meenakshi Mukerji’s compound of five octahedra (inspired by Dennis Walker) is also a bit fally-aparty, but I like it as – unlike many of these models – it genuinely is the polyhedron so-named, rather than something where you have to squint at the holes in the wire-frame and imagine faces there.
![Compound of five octahedra [CC-BY-SA-3.0 Steve Cook]](https://www.polypompholyx.com/wp-content/uploads/2017/01/compound_of_five_octahedra-300x288.jpg)
Compound of five octahedra. You can easily see the yellow octahedron here: the sixth spike is underneath the model; the other four colours are similarly interlaced.
The
five intersecting tetrahedra are actually a lot easier to make than they look.
Francis Ow’s 6-degree modules themselves are easy to fold, and the vertices are a lot more robust than they might appear. The most difficult bit is getting the modules interlinked in the right way. I’ve managed it twice, but only whilst staring at the YouTube video and performing assorted “purple = green” gymnastics in my head.
![Compound of five tetrahedra [CC-BY-SA-3.0 Steve Cook]](https://www.polypompholyx.com/wp-content/uploads/2017/01/compound_of_five_tetrahedra-300x291.jpg)
Compound of five tetrahedra – party piece.
Michał Kosmulski’s page has lots of lovely illustrations, instructions and inspirations. I found
Tung Ken Lam’s blintz icosadodecahedron (also credited as
Francesco Mancini’s UVWXYZ intersecting planes model) there. It has the same symmetry as the Electra icosadodecahedron above, but you can see the six intersecting pentagons more clearly. Both have the same underlying structure as the
Hoberman sphere – that expanding/contracting plastic stick model thing beloved of science fairs.
![UVWXYZ intersecting plane icosadodecahedron [CC-BY-SA-4.0 Steve Cook]](https://www.polypompholyx.com/wp-content/uploads/2017/01/intersecting_plane_icosadodecahedron-300x278.jpg)
UVWXYZ intersecting plane icosadodecahedron
This last one is a bit of a cheat as (in theory, and mostly in practice too) the structures above are held together by nothing more than friction. Valentina Gonchar’s
revealed flower star kusudama has to be glued, which is kind-of cheating, but I couldn’t resist as it is two structures in one:
![Flower star [CC-BY-SA-3.0 Steve Cook]](https://www.polypompholyx.com/wp-content/uploads/2017/01/flower_star-300x153.jpg)
Revealed flower star – shut (left) and popped open (right).
Things I’d still like to do:
- Build a much larger PhiZZ ball (270 units): this would be useful for demonstrating the structures of viral capsids. UPDATE: Done!
![270 PHiZZ knolled parts [CC-BY-SA-3.0 Steve Cook]](https://www.polypompholyx.com/wp-content/uploads/2017/01/phizz_270_knolled-225x300.jpg)
Before…
![270 PHiZZ [CC-BY-SA-3.0 Steve Cook]](https://www.polypompholyx.com/wp-content/uploads/2017/01/phizz_270-300x300.jpg)
…After
- I’ve not yet found a good great dodecahedron model: they exist on Pintrest, but I’ve yet to find any instructions for one. UPDATE: Done! (Couldn’t for the life of me work out how to do 3-colouring, but module is from Saku B, recommended by Nick in the comments below)
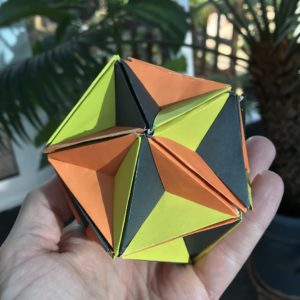
Great dodecahedron
- I have lost wherever it was I found the instructions for this inwardly cumulated rhombic triacontahedron: I’d quite like to rediscover them so I can credit the inventor! UPDATE: this isn’t where I originally saw it, but AresMares by Gewre has a video tutorial, and a kind commenter has let me know the designer is Silvana Betti Mamino – thank you!
![Rhombic triacontahedron [CC-BY-SA-3.0 Steve Cook]](https://www.polypompholyx.com/wp-content/uploads/2017/01/rhombic_triacontahedron-300x288.jpg)
Rhombic triacontahedron of unknown source.